Post Syndicated from original https://www.toest.bg/na-vtoro-chetene-cyaloto-nashe-bezumstvo/
„Цялото наше безумство“ от Ши-Ли Коу
превод от английски Светослава Павлова, изд. „Алтера“, 2022
Обикновено има или целенасочен интерес и изрично любопитство, или някаква странна съпротива и съмнение, когато човек посяга към литература от автори, принадлежащи на крайно далечна от нас култура. Признавам си, че зачетох „Цялото наше безумство“ на малайската авторка Ши-Ли Коу по втория начин само за да установя
колко изумително сходен на нашия е светът, който тя описва –
в устройството на обществото и специфичните му недъзи; в хитрите и често смехотворни преки към справянето с бита, спецификата на отношенията и живописните характери в провинцията; в противопоставянето на глобалния мегаполис и справянето с неумолимо настъпващия „прогрес“; в шегите и езика.
Романът представлява двугласен хор от разкази в първо лице, като едното повествование се води от възрастен етнически китаец, а другото – от подрастващо християнско момиче.
Съдбата на двамата се преплита, когато момичето бива осиновено след катастрофа от близката на мъжа – малайката Мами Биви. Жена, която като че ли сме срещали в редица други любими книги: самодостатъчна особнячка, самотна и самобитна, чудата и доизмисляща истории, всяка от които започва с „Беше/Имаше неестествен…“. Тази дума – неестествен, ще бележи всичко случващо се в измисления градец Лубок Сайонг в щата Перак, така че дори нормалните и обичайни неща ще придобият някакъв друг, извънвремеви и леко магичен привкус. Така както някои от героите ще виждат неспокойни мъртви или ангели, рибите ще имат съзнание, а част от събитията ще се случват като че ли по неведомо съвпадение.
Не, романът далеч не е в традицията на латиноамериканския магически реализъм, макар тук-там да има подобни краски.
Разказът е изключително земен, изпълнен със сладостна ирония и ярък социален сарказъм.
Книгата не представлява също така нито мемоари на възрастния мъж (макар и донякъде да наподобява градски летописи, водени в ние-форма), нито роман на израстването в аз-форма, както се споменава в анотацията. Може би защото героите разказвачи не са наистина важните, фокусът не е тяхната промяна или израстване, те не са в центъра на събитията.
Големият герой на този роман е всъщност самият градец, както и неговите типажи, чешити, местни и случайно приходящи. Това неголямо и не особено уредено място няма как да не ви се стори безкрайно познато – то все още е далеч от инфраструктурата, привилегиите и шаблонния лукс на глобалното, макар „прогресът“ по един или друг начин да пълзи към него. Иска ли питане, че политиците минават през него инцидентно преди избори и с известна погнуса, а ежегодните наводнения са нещо, което жителите са приели като природна даденост, както – уви – често се случва и у нас:
В Лубок Сайонг не виним пътното строителство, обезлесяването, затинените реки, запушените отводнителни тръби или универсалния виновник, корумпираните бюрократи, които работят в отделите, отговарящи за гореспоменатите пътища, гори, реки и тръби.
В този град, където всичко е едно – една болница, едно полицейско управление, една бензиностанция и така нататък – легендата е най-близката възможност за преодоляване на нелицеприятната и скучна истина за реалността такава, каквато е. Дори и тя обаче е оскъдна и не особено атрактивна, за да привлича достатъчно интерес към града, или както разказвачът описва местното предлагане на легендите –
хладни и слабо гарнирани, на порции, недостатъчни да задоволят апетита или въображението.
Ши-Ли Коу е изключително забавна в начина, по който кара разказвача си да (само)иронизира местните поверия, да „героизира“ на шега обикновеното, докато подкача „автентичното“ и „традиционното“, да извежда на преден план смешното, като същевременно буди у нас симпатия към него.
Съпоставяйки от време на време живота с този в столицата Куала Лумпур, авторката очертава ясни дихотомии, без нито за миг да бъде мелодраматична или да идеализира едното за сметка на другото: уж автентичното ръчно труд-и-творчество (често „подпомагано“ от поръчаните в Китай масовки) срещу индустриализацията и големите фабрики, в които в крайна сметка работят същите „мързеливи, глупави и злочести хора, които просто се опитват да си изкарат прехраната“; неугледния локален туризъм, на който се отдават само случайно изпаднали авантюристи, срещу маститите спа комплекси за добре плащащите чуждестранни гости; местната храна, която може да предизвика позиви за често ходене до тоалетната, срещу безличните световни вериги, като „Старбъкс“ и „Макдоналдс“; самодоволните доброволци от големия град и свикналите с бедствията провинциалисти, които се дразнят от тяхната жизнерадостна и клиширана помощ; забавната неприветливост на самобитното срещу абсурда на привнесеното; традиционните ислямски закони и илюзорното приличие срещу реалното съществуване на травестити и други сексуални и личностни свободи зад кулисите.
Възможно е тогава да се каже, че „Цялото наше безумство“ може да се чете като своеобразна социална сатира,
тъй като във всяка възможна ситуация и чрез куп метафори Ши Ли-Коу не пропуска да ни напомни за обществените реалности. Макар и история на един отделно взет малък град, тя е история на съвременна Малайзия встрани от лъскавите туристически брошури. Ши Ли-Коу очертава ежедневието на земния, обикновен човек (чиято практичност измества дори въпросите на живот и смърт) чрез поредица от забавни, полукомични и леко тъжни случки. Ще научим как се клинчи от час, как се спасяват пиявици, как се превъзпитават травестити, как се прави мост в нищото и т.н. И във всичко това ще личи носталгията по непревзетото от глобализма, по малкия град, по твърде човешкото.
Тази книга наистина е изпълнена с неподправено човеколюбие,
с великодушно снизхождение към кривиците и недостатъците на всеки и с обич към доброто и достойното у всеки – независимо от неговата етническа, верска, сексуална и друга принадлежност. В мешавицата от етноси, която Ши-Ли Коу забърква – малайци, индийци, китайци, индонезийци, – човекът в която и да е координатна точка на споменатото в горния абзац остава просто човек: погрешим, в никога незавършен процес на себесъздаване, очарователно комичен. Важното е, че той не е сам за себе си – героите на романа неизбежно намират път един към друг, колкото и криволичещ да е той, образуват сложни, но автентични връзки и в крайна сметка една истинска човечна общност в епруветката на измисления Лубок Сайонг.
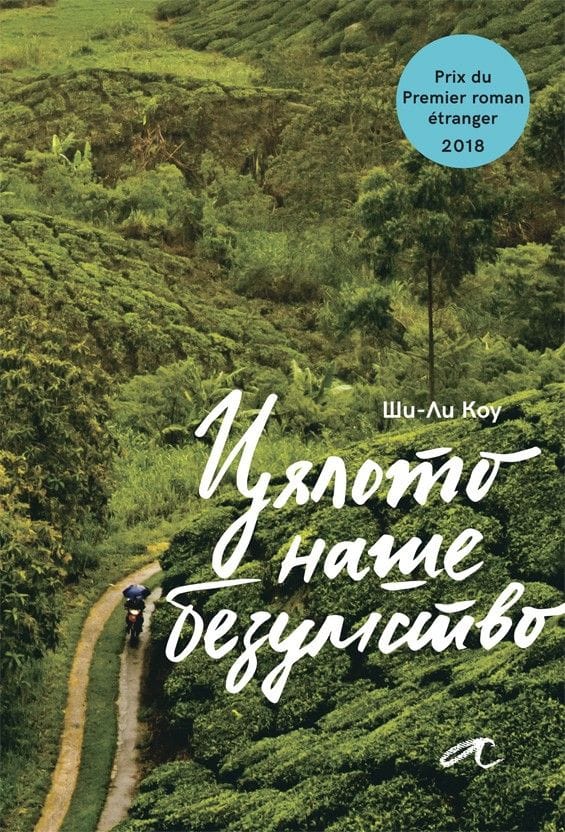
В „Цялото наше безумство“ няма поука, както признава чрез героите си авторката:
Искам да кажа на тези, които идват, на всеки, който би ме чул, че урокът не е в разказа, а в живота.
И за да затворим кръга към далечното, което особено на нас, българите, ще ни се стори безкрайно познато, ще ви оставя
кратък списък с някои неща от ежедневния дискурс, които веднага ще разпознаете като родни
и които присъстват в книгата:
- всичко е политика;
- таксиметровите шофьори се оплакват, че държавата е съсипана и никой не се грижи за тях, а всички искат да ги прецакат;
- всичко гнило идва от Китай – отровено мляко за кърмачета, консервирана храна с живак, масово произведените сувенири;
- всички гледат ужасно дублирани сапунени опери;
- хората в крайна сметка си предпочитат съмнителната и мързелива помощ на корумпираните институции;
- те споделят тихото чакане и кротката търпимост по отношение на некадърността, защото общата мизерия свързва;
- политиците раздават пари на калпак преди избори на всички социално ощетени групи освен данъкоплатците на платена работа;
- природните бедствия са по вина на корупцията и нехайството, но са представяни като добри за нацията, защото карат обществото да се обединява около обща кауза;
- младите, които все пак остават в малкия град, просто твърде много обичат мързеливия си живот, за да се бъхтят на някаква работа;
- прогресът не е исторически, а преди всичко търговски и се измерва с потреблението на куп жалонни боклуци.
Завършвам с една присъда на Мама Биви:
Вие сте тези, които погубихте Куала Лумпур. Продължихте да строите магазини, офиси, жилищни сгради и огромни молове. Живеехте си в множеството ви мезонети и вили и те пак не ви бяха достатъчни. Строите още по-големи сгради, за да продавате все повече неща. Настоявате за все повече чуждестранни майстори готвачи и лъскави ресторанти, които трябва да се обновяват на всеки три години. Построихте още пътища за шестте ви коли, с които задушавате града, защото много ще се изпотите, ако се качите в метрото или автобуса. И се оплаквате, че градът бил загубил чара си?
Никой от нас не чете единствено най-новите книги. Тогава защо само за тях се пише? „На второ четене“ е рубрика, в която отваряме списъците с книги, публикувани преди поне година, четем ги и препоръчваме любимите си от тях. Рубриката е част от партньорската програма Читателски клуб „Тоест“. Изборът на заглавия обаче е единствено на авторите – Стефан Иванов и Антония Апостолова, които биха ви препоръчали тези книги и ако имаше как веднъж на две седмици да се разходите с тях в книжарницата.